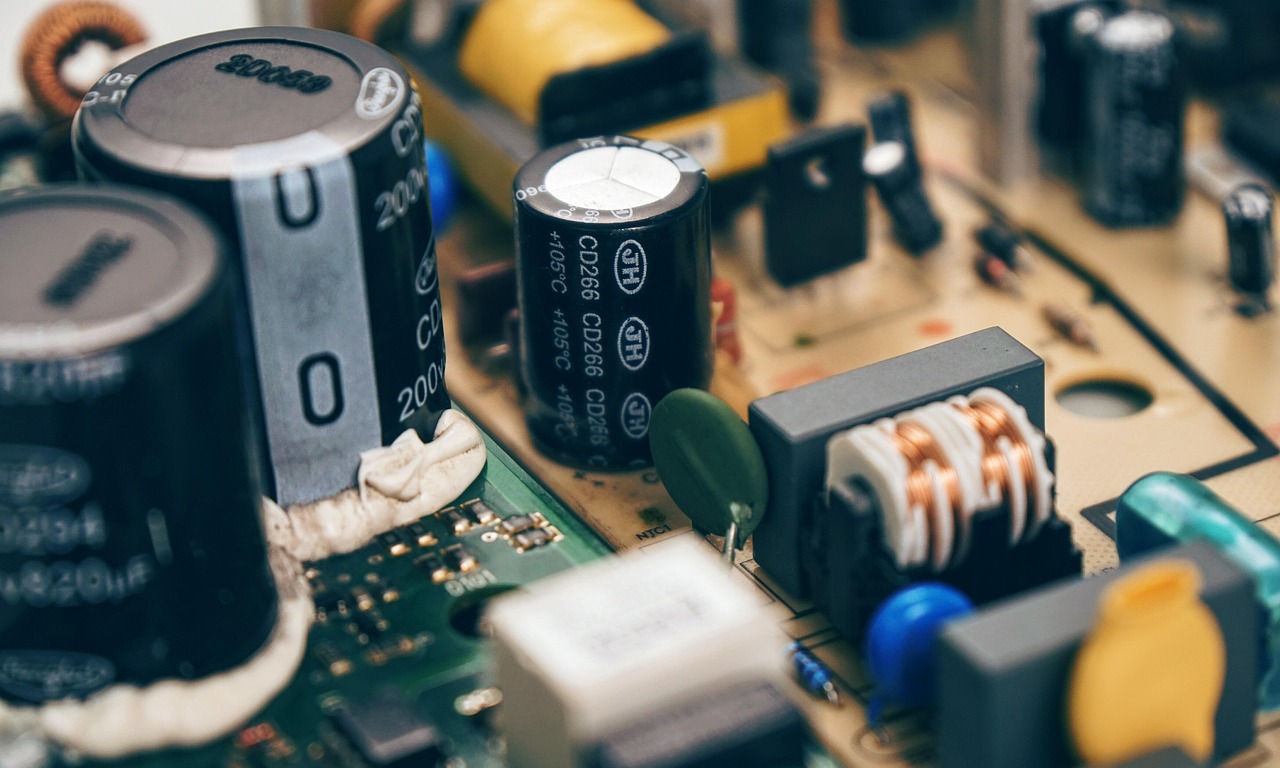
Choosing capacitors for your next application comes with many considerations, including environmental, electrical, and mechanical factors. In this guide, we will explore these considerations, the types of capacitors that exist, and why choosing the right one is so important.
The Importance of Consideration in Choosing Capacitors
Capacitors. They're the unsung heroes of the electronic world, quietly doing the heavy lifting when it comes to storing and releasing electrical energy. You’ll find them in everything from your phone to industrial machinery, in roles that range from filtering to smoothing power supply voltages. But despite their seemingly humble appearance, these little components can make or break an entire circuit, and, frankly, they often do.
In the controlled environment of a lab, choosing a capacitor based on a schematic’s specifications is pretty straightforward. You’ve got your requirements laid out, you pick a capacitor, and you move on. In such a setting, the environment is largely stable, and the capacitor has the luxury of operating within narrow parameters. But, let’s not kid ourselves—real-world applications don’t operate in a vacuum (literally and figuratively).
For instance, consider automotive systems. The vibrations, the extreme temperature fluctuations, the moisture... these aren't exactly the most welcoming conditions for capacitors that were designed for use in your office desk setup. What works in the clean, controlled environment of a lab could fail spectacularly when thrown into the harsh reality of an engine compartment or under the hood of a car, where heat can rise to a sweltering 100°C, moisture can wreak havoc, and vibrations can shake even the most stable of components loose. Choosing the wrong capacitor for such an environment? That's a disaster waiting to happen.
It might seem obvious, but let’s make it crystal clear: choosing the wrong capacitor does not just mean your circuit might stop working. Oh no, it’s much worse than that. From an engineering perspective, a capacitor failure could lead to a malfunction or even a complete breakdown of the device. Depending on the application, this could mean everything from a failed product launch to a full-on system crash. And we’re not even talking about the worst-case scenarios yet.
On the legal side, things get even stickier. If a circuit failure caused by a capacitor leads to damage—whether it’s to surrounding equipment, a loss of data, or, heaven forbid, harm to individuals—who do you think gets the blame? That’s right, the engineer who chose the wrong capacitor. No one’s going to care that it worked fine in your lab or office. You’re the one who signed off on the design, and you could be held liable for all the consequences that follow. Lawsuits, fines, and damage to your reputation can be more expensive than you ever thought possible.
So, how do you avoid all this? How do you choose the right capacitor for the job? It’s all about considering the real-world environment in which the capacitor will operate. In fact, engineers must go beyond just basic schematic requirements and take into account factors like temperature tolerance, vibration resistance, and the potential for moisture exposure. Selecting the right capacitor isn’t just about picking a number from a datasheet—it’s about ensuring your design can withstand the conditions it will face in the field.
Electrical Considerations for Capacitors
When it comes to selecting a capacitor, the first thing that should grab your attention is the electrical requirements. Now let's not sugarcoat this: getting the electrical specifications wrong is one of the quickest ways to make sure your design fails. Whether you’re smoothing power in a high-current motor driver or just handling a bit of decoupling for a low-power microcontroller, the electrical characteristics of a capacitor are non-negotiable.
Capacitance Value
The first thing we all look at is capacitance, measured in farads (or often microfarads or picofarads in the case of smaller values). It’s the basic measurement of how much energy a capacitor can store. Too much capacitance, and your circuit could suffer from sluggish response times or even unwanted delays in signal processing. Too little, and your system might experience voltage dips, noise issues, or instability. Picking the right capacitance is easy if you understand your circuit's needs—just remember that it’s a balancing act.
Voltage Rating
Voltage rating, however, is where things get a little more interesting, and potentially dangerous if you're not careful. Simply put, if the voltage rating of a capacitor is too low for the operating voltage in the circuit, you're asking for a disaster. When the voltage rating is exceeded, dielectric breakdown occurs, and the capacitor can fail catastrophically, sometimes even resulting in an explosion. No one wants to deal with that, and frankly, it’s a rookie mistake you should never make. Always choose a capacitor with a voltage rating that far exceeds the highest voltage your circuit will ever see (at least a 50% safety margin).
Equivalent Series Resistance (ESR)
Next up is Equivalent Series Resistance (ESR), a crucial spec that’s easy to overlook. ESR represents the internal resistance of the capacitor, and it can have a huge impact on the efficiency of your design. High ESR can result in excessive heat build-up, power losses, and poor performance, particularly in high-frequency applications or circuits that demand high ripple current. Low ESR is generally desired in most power supplies and signal smoothing applications because it ensures the capacitor performs efficiently and without unwanted heat generation. But keep in mind, the acceptable ESR range varies based on the application—an electrolytic capacitor meant for bulk power filtering might tolerate higher ESR than one used for signal integrity in high-speed circuits.
Ripple Current Capacity
Speaking of high-frequency applications, let’s talk about ripple current. Ripple current is the AC component that flows through a capacitor in addition to the DC current, and it’s particularly relevant in power supplies. If the capacitor can’t handle the ripple current, it will overheat, degrade quickly, and eventually fail. So, if you're working with anything like a motor driver or power regulation circuits, be sure to check the capacitor’s ripple current rating. If it’s too low, you're asking for trouble. A capacitor that can’t handle high ripple current will quickly become a weak link in your system, leading to instability or failure.
Tolerance
Capacitors also come with tolerances that need to be considered carefully. Tolerance refers to the allowable variation from the nominal capacitance value, and it’s critical in applications where precision is key. For example, if your circuit is dependent on timing or signal processing, such as in oscillators or filters, you need a capacitor with a tight tolerance. A ±20% tolerance might be just fine for basic decoupling or bulk filtering, but in high-precision tasks, you’ll want something tighter—think ±1% or even better. The tighter the tolerance, the more precise the component will be, and the more stable your circuit will perform. So, don't go thinking that a wide tolerance range is always acceptable; sometimes, it’s the difference between success and failure.
Thermal Considerations for Capacitors
When you’re choosing the right capacitor for your design, the electrical characteristics are only just the start of. Heat is a silent killer in electronics, and if you’re not paying attention to how your capacitor will behave under operating conditions, you might end up with a ticking time bomb.
Temperature Sensitivity
Capacitors, like all electronic components, are highly sensitive to temperature. This isn’t just a theoretical concern—temperature affects the very core of a capacitor’s performance. Even if you’ve selected the perfect capacitor in terms of capacitance and voltage, it’s the heat generated from continuous operation (and the surrounding environment) that could mess things up. If you're not considering the thermal effects, you're leaving yourself vulnerable to performance degradation and even catastrophic failure.
Thermal Coefficient and Its Impact
The effect of temperature on capacitors is typically captured by the thermal coefficient. This essentially means that as the temperature increases or decreases, certain characteristics of the capacitor, such as its Equivalent Series Resistance (ESR) and leakage current, will also change. You may think that’s just a minor detail, but believe me, it’s not. For example, when a capacitor’s ESR increases due to heat, its efficiency drops, and that leads to higher heat generation, creating a vicious cycle (called thermal runaway).
Electrolytic Capacitors: The Hotter They Get, The Worse They Perform
Electrolytic capacitors, in particular, are far more sensitive to temperature changes than their ceramic or film counterparts. If you're working with electrolytics in high-power or high-frequency applications, you need to be extra cautious. These components are known for having a shorter lifespan when exposed to heat, and they also tend to have higher ESRs, especially as temperature rises. Even slight increases in temperature can significantly impact their performance, shortening their operational life and affecting the overall reliability of your circuit.
Heat and Ripple Current: A Dangerous Combination
Capacitors used in power smoothing applications need to handle significant AC ripple in addition to the DC voltage. The issue here is that as the ripple current increases, the heating effect inside the capacitor also rises. As the capacitor approaches its maximum voltage and ripple current ratings, the internal heating becomes more pronounced, which can lead to degraded performance, reduced lifespan, or total failure. This is where your capacitor’s temperature rating becomes crucial. If you're not choosing one with an adequate thermal margin, you're setting yourself up for failure.
Impact on Current Handling and Longevity:
Temperature doesn’t just affect ESR—it can also reduce a capacitor’s maximum current handling capability. Consider this: a capacitor rated for 1000mA at room temperature (about 25°C) may only be able to handle 700mA or less at its maximum operating temperature (typically between 85°C and 125°C). That’s a substantial drop, and in some designs, it could mean the difference between a perfectly functioning system and one that’s constantly overheating or shutting down due to overcurrent conditions.
Mechanical Considerations for Capacitors
Another equally important consideration that must be made are those involving the mechanical design of capacitors. The size, form factor, and even the weight of the capacitor can have a substantial impact on your circuit, particularly in today’s world of miniaturized and highly efficient designs.
Physical Size and Circuit Layout
The physical size of a capacitor can drastically affect the overall size of your design. For large, space-abundant applications, you might not think twice about the size of a capacitor, but as designs get smaller and more compact, it becomes a critical factor. Capacitors come in a variety of package sizes, and your choice can either enhance or limit your design’s potential.
For instance, larger capacitors such as those in radial or through-hole packages offer more flexibility, typically allowing higher capacitance values and easier handling during assembly. These are great for larger systems or prototypes where space is less of an issue. However, in smaller, more compact designs—think wearable devices, smartphones, or IoT devices—the physical size of the capacitor becomes a critical factor in the overall layout.
In the era of miniaturized electronics, smaller SMD capacitors (like 0805, 0603, or even 0402 and 0201) are the real heroes. These small, flat packages allow for high-density placement of components, enabling designs to shrink down significantly. When you’re designing something like a smartwatch or a wireless earbud, these tiny capacitors can make or break the size constraints of your design.
But don’t be fooled by their small footprint—just because a capacitor is tiny doesn’t mean it should be chosen blindly. You still need to consider electrical properties, voltage ratings, and other factors, but the mechanical size can be a game-changer in tight spaces.
Low-Profile Designs
Aside from the length and width of capacitors, the height (or thickness) is another crucial factor in design. The height can be just as significant as the footprint when you’re trying to design low-profile, slim devices. Every millimeter counts, especially in consumer electronics, where sleek, slim designs are often a top priority.
A capacitor that’s too tall could prevent your design from achieving the necessary thickness, potentially forcing you to rethink your entire layout or, worse, abandon an essential feature. Always keep height in mind, particularly when designing for wearables, portable devices, or applications where compactness is critical.
Weight Considerations
Weight might not always be at the forefront of your mind, but it can be a crucial factor depending on your application. For example, when dealing with large household appliances like microwaves or washing machines, weight isn’t generally an issue.
However, in lightweight, high-performance applications such as drones or electric vehicles (EVs), every gram matters. Capacitors, especially when used in large quantities, can contribute to the overall weight of the device. In these applications, weight adds up quickly and can directly impact performance, battery life, and even aerodynamics.
For drones, the weight of capacitors can influence how long the battery lasts and how well the drone performs in the air. In electric vehicles, weight can affect battery efficiency and overall range.
So, if you're designing for these types of applications, it’s important to consider the weight of the capacitors you're choosing and how it impacts the final design.
Material Considerations for Capacitors
When you're designing circuits that need to just work—every time, under every condition—you can't afford to ignore the materials your components are made of. And capacitors? They're the poster child for material-driven performance differences. While the capacitance value might be printed boldly on the body, the real story lies in what’s inside. Different dielectric materials dramatically influence behavior, longevity, and reliability. If you’re selecting capacitors without considering their construction materials, you’re basically designing with a blindfold on.
Electrolytic Capacitors: Bulk with Baggage
Electrolytics are the go-to for high-capacitance applications, particularly in power supplies and DC bulk storage. These use a liquid (or solid) electrolyte and aluminum or tantalum electrodes, which gives them their high capacitance density.
However, that comes with a price:
-
Higher ESR: Which means more heat and power loss, especially at high frequencies.
-
Leakage current: Particularly in lower-quality variants, this can become a design liability.
-
Aging: Electrolytic capacitors tend to dry out over time, especially in hot environments, reducing their effective capacitance and life span.
Still, in many power applications, there’s just no getting around the sheer capacitance value they offer per dollar and per cubic millimeter.
Ceramic Capacitors: Small, Cheap, and Sometimes Tricky
Ceramics are the Swiss Army knife of the capacitor world. You’ll find them everywhere—from decoupling logic chips to RF circuits. They have low ESR, low leakage, and come in a wide range of sizes and capacitances.
But beware: the type of ceramic matters a lot.
-
Class I ceramics (like C0G/NP0): Extremely stable, low loss, and perfect for precision circuits.
-
Class II and III ceramics (like X7R, Y5V, Z5U): Higher capacitance, but with trade-offs. These materials can suffer from temperature drift, voltage coefficient issues, and even physical aging—yes, they lose capacitance just sitting on a shelf.
For digital decoupling, that’s usually acceptable. But in analog, audio, or timing applications? Not so much.
Film Capacitors: Big, Stable, and Reliable
Film capacitors (polyester, polypropylene, PTFE, etc.) are known for rock-solid stability. They don’t drift much with temperature or time, and they have excellent electrical noise performance. You’ll see them in:
-
High-fidelity audio gear
-
Precision analog circuits
-
High-voltage filtering
They're generally bulkier than ceramics for the same capacitance, but when stability and signal integrity matter, film caps are hard to beat.
Tantalum Capacitors: Premium Performance, Premium Risk
Tantalum capacitors offer excellent capacitance in compact packages and are often used where stable, long-term performance is critical—like in aerospace or medical devices. But there’s a caveat: if mistreated (reverse voltage, overvoltage, etc.), tantalums don’t fail gracefully. They can fail catastrophically. Think smoke, sparks, and a really bad day in the lab.
That said, when used correctly with proper derating, they’re incredibly reliable and long-lived.
Application-Specific Materials: Beyond the Basics
In harsh environments like aerospace, defense, or automotive, capacitor materials must meet stricter demands. Think flame retardance (UL94 compliance), extreme temperature tolerance, and chemical resistance. It's not just about the dielectric anymore—case materials, encapsulants, and terminations all come into play.
For example:
-
High-temp automotive environments may call for ceramics rated to 150°C+ with anti-vibration packaging.
-
Aerospace-grade film capacitors might be housed in flame-retardant epoxy with MIL-SPEC ratings.
-
These aren’t off-the-shelf parts from the bargain bin. You pay more, but you also sleep better.
Environmental Considerations for Capacitors
You can design the perfect circuit in a lab, simulate it flawlessly in SPICE, and prototype it with precision—but once that design leaves the bench and enters the real world, Mother Nature has her own agenda. And if you haven't chosen components that can handle the conditions they'll face, your "perfect" design is going to fail—quietly or catastrophically.
Capacitors are no exception. These humble two-terminal components are surprisingly sensitive to the environment around them. From humidity and temperature to mechanical stress and radiation, external factors can dramatically affect performance and lifespan. Ignoring this part of the selection process isn’t just bad engineering—it’s asking for failure.
Moisture, Humidity, and Corrosion
Moisture is the silent killer of many capacitors—especially electrolytics. Their internal electrolyte can degrade when exposed to high humidity or condensation, leading to corrosion of internal electrodes and leakage over time. If the capacitor isn't hermetically sealed (and most aren’t), expect performance to degrade faster in damp environments.
Outdoor or industrial settings? Use capacitors with conformal coatings or hermetic seals. Long-term reliability required? Avoid low-grade electrolytics in anything exposed to environmental moisture.
A single drop of water inside a capacitor can turn into an internal short, a slow chemical reaction, or an outright explosion if it hits high voltage. That’s not theoretical—it’s seen often in the field.
Temperature Cycling and Thermal Fatigue
Constant thermal expansion and contraction—what engineers refer to as thermal cycling—is another major killer. This is especially true in environments with wide temperature swings, such as automotive engine compartments or outdoor enclosures.
For example, dielectric materials can develop microcracks under repeated stress, solder joints and terminations can fatigue, especially in SMD capacitors with mismatched thermal expansion coefficients, and cracks in MLCCs, in particular, can cause shorts or intermittent failures, which are some of the hardest issues to troubleshoot post-installation.
Pressure and Chemical Exposure
In environments such as industrial plants, food processing facilities, or medical labs, exposure to vapors, cleaning agents, and other chemicals can be problematic. Capacitor casings and terminations can degrade or react with aggressive chemicals—especially in the case of cheaper plastic-encased devices.
Capacitors used in these environments often need to comply with RoHS, REACH, and even FDA material compatibility standards. It’s not just about electrical specs—it’s about the whole system being chemically and biologically safe.
Shock and Vibration
If your design moves—literally—you need to plan for mechanical stress. This includes automotive ECUs, drones, aerospace and aviation systems, and heavy industrial machinery. Sustained vibration or sudden shocks can cause lead fatigue, cracked casings, or fractured dielectric layers. Use capacitors with vibration-resistant terminations and, where possible, opt for flexible terminations or board-mount adhesives to help mitigate physical movement.
Radiation and High-Energy Environments
Extreme cases like spacecraft, satellites, or nuclear applications introduce a whole new layer of complexity. Radiation-hardened (rad-hard) capacitors are built with specialized materials and tested to resist degradation from high-energy particles.
For example, standard ceramic capacitors may depolarize or change value in radiation-heavy zones, while electrolytics are not even considered in these applications (being far too sensitive). Because of the unique nature of such environments, capacitor selection becomes almost a topic of materials science and physics more than just engineering.
Supply Chain Considerations for Capacitors
Capacitors are one of those quiet heroes in electronics. They don’t get the glamour of a microcontroller or the mystique of a GaN transistor, but without them, most electronic systems would collapse faster than a government budget. They’re everywhere—filtering, coupling, decoupling, timing—and because of that, they’re often treated as an afterthought in the sourcing process. That’s a mistake.
When your design includes a few dozen (or a few hundred) capacitors, the part selection process isn't just about ESR and capacitance values anymore. Suddenly you're balancing cost, availability, lifecycle, and sourcing reliability—especially when you're building something at scale. Cheap caps from a mystery vendor might work fine for a weekend bench project, but in the real world—where products ship in thousands and recalls cost millions—you need to do better.
Compliance
Let’s start with compliance. In regulated industries like automotive or aerospace, the part's electrical specs are just the tip of the iceberg. You also need supplier traceability, documentation of the manufacturing process, and certification under systems like ISO 9001 or IATF 16949. That “bag of caps” from the discount online shop? Good luck getting a Certificate of Conformance out of them, let alone a full material disclosure or traceability to the original wafer lot.
Quality Control
Even if you’re not working in a heavily regulated industry, these quality systems matter. Why? Because even in consumer electronics, a failure in the supply chain can shut down your production line. Supply issues can range from genuine shortages (hello, MLCC crisis) to something worse: counterfeit parts. These aren't just fakes—they can be catastrophic. A counterfeit capacitor might pass initial test, only to fail prematurely in the field, leading to product failures, warranty claims, and a massive hit to your brand's reputation. And once that’s gone, good luck getting it back.
It’s also worth noting that not all “equivalent” capacitors are actually equivalent when you look under the hood. Two capacitors might match in value and rating, but vary wildly in terms of performance under ripple current, temperature stability, or lifetime. Worse still, some suppliers might silently change part specifications mid-production due to material shortages, meaning your reliable cap suddenly isn't so reliable.
The lesson here is simple: sourcing capacitors is not a checkbox—it’s a strategic decision. You need to qualify suppliers, demand documentation, and build relationships with vendors who understand that consistent, traceable quality isn’t optional. It’s a requirement.
Choosing a Good Supplier for Capacitors
So you’ve dialed in the specs—voltage rating, capacitance, ESR, maybe even checked the ripple current curves like the diligent engineer you are. Now comes the fun part: sourcing the thing. And while it might be tempting to treat capacitor sourcing like a price-per-unit arms race, let’s be clear—where you get your parts from matters just as much as what you get.
Reputable Suppliers
A good capacitor supplier isn’t just someone with stock—they’re your first line of defense against counterfeit parts, spec drift, supply chain interruptions, and even legal headaches. The best ones aren’t necessarily the cheapest, but they are the ones who’ve been around long enough to know what “quality control” actually means. Years in the industry, long-standing relationships with OEMs, and a portfolio of satisfied, returning customers are strong indicators that they know how to deliver components that meet real-world requirements—not just the line items on a datasheet.
R&D Purchasing vs. Production
When you’re still in the prototyping phase, you might get away with buying from a lesser-known or newer supplier. That’s your sandbox—experiment, iterate, have fun. But when you shift into production, especially at volume, that’s when you need to lean on suppliers with a rock-solid reputation. These companies aren’t just selling you parts—they’re offering you consistency, documentation, and most importantly, accountability.
Legal Implications
Speaking of accountability, here’s a cold hard truth: big-name suppliers have lawyers. They have customer service reps. They have RMA processes. If a batch of capacitors fails to meet spec or shows signs of manufacturing defects, you’ve got a clear path to recourse. You might not like having to go through it, but it’s there. Contrast that with a fly-by-night distributor or some guy with an AliExpress storefront and a Gmail contact address. When something goes wrong there—and it will—you’re out of luck and out of money.
And sure, you might save a few cents per unit by going with a no-name vendor. Multiply that by a few thousand units, and maybe it looks like a good deal. But when those parts cause a recall, lock up a production line, or worse—fail in the field—that short-term saving becomes a long-term financial sinkhole.
Bottom line? Play it safe. Stick with reputable, vetted, and certified suppliers. Pay a bit more up front if you have to. Because when it comes to sourcing capacitors, you’re not just buying parts—you’re buying peace of mind.
Making the Right Call on Capacitors
At a glance, choosing a capacitor might seem like the simplest part of your design—just slap in a 0.1 µF ceramic and call it a day, right? In a lot of cases, sure. The humble SMD ceramic cap is the backbone of modern electronics for a reason. But if you’ve read this far, you already know it’s not always that simple.
Every capacitor in your design plays a role—whether it’s filtering noise, stabilizing voltage, or handling transient loads—and if one of them fails, it can drag the whole system down with it. That’s why it's not just about which capacitor you pick, but how you pick it—and where you get it from.
This guide isn’t just about specs and sourcing—it’s about thinking like a professional. When you understand the electrical, thermal, mechanical, and environmental demands of your design—and how supply chain realities affect your component choices—you’re not just selecting parts. You’re engineering for reliability, performance, and long-term success.
So next time you’re digging through a BOM or spec sheet, don’t treat capacitors like filler. Treat them like the critical components they are. Because in real-world engineering, the “small stuff” can absolutely make or break your design.